By Colleen Zewe
Medill Reports
Have you ever had an electrical current sent from your brain to your arm to research strength? Surprisingly, it doesn’t hurt – it’s done for the sake of neuromuscular research at the University of Pittsburgh School of Health and Rehabilitation Sciences Neuromuscular Research Lab.
The lab uses a magstim rapid transcranial stimulator, called a TMS for short. The TMS machine uses copper wires to send electrical signals through the skull, creating an electrical signal to the intended muscle. When I had a current sent through my body, it felt like being tapped on the knee for a reflex test.
Doctoral student Anne Beethe uses these electrical signals in her research to study the bilateral deficit phenomenon. It is a big term explaining some common experiences. Weightlifters: Have you ever noticed that you can lift 100 pounds with your right or left arm, but when you try to lift 200 pounds with both arms at the same time, you can’t as easily lift all that weight? This week, I learned more about Anne Beethe’s research on the bilateral deficit phenomenon, or the idea that bilateral movement is more difficult than unilateral.
In this video, you can see the subject’s arm twitching when Beethe sends an electrical current through his brain.
Beethe used the example of toddlers to describe how the bilateral deficit develops. “Have you ever been around toddlers? Do you notice how they move bilaterally, and they kick bilaterally?” she asked. For example, a toddler sitting in a high chair may move both arms at once while eating, or kick their legs simultaneously.
Beethe said that is because they have not yet reached full function of their corpus callosum, which is made up of nerve fibers that run right between the two hemispheres of the brain. The fibers connect the two sides of the brain, but also allow the two hemispheres to act independently. Once the corpus callosum is fully grown and the two hemispheres are separated, the left hemisphere of the brain controls movement on the right side of your body, while the other controls movement of the left.
The corpus callosum develops while a baby is still in the womb, but the fibers do not reach their maximum functionality until early adolescence. In early childhood, the fibers get stronger and stronger. So, at a young age, babies do not yet have the ability to control only one side of the body. Eventually, however, the corpus callosum becomes so strong that unilateral movements, or using only one arm or leg at a time, becomes natural. That’s why we can write, pick things up and toss a ball with one arm.
Beethe hypothesizes that the separation of the two brain hemispheres makes it difficult for the brain to send signals to both arms simultaneously when lifting heavy loads. While we can use both limbs for easy, routine tasks like typing, tasks with more muscle contractions require more work from the brain. For her dissertation, Beethe is looking at the neurological signals the brain sends. She compares the signals when they are sent to just one arm versus simultaneously to both. These signals could point to why it’s hard for athletes to produce maximal force using both arms at once. If they have always trained with unilateral movements, it can be hard for the brain to adjust to bilateral movements, because it requires the two sides of the brain to work at the same time and compete against each other.
“Whenever we move, since we have two motor cortices, one likes to take over,” Beethe explained. “In order for me to move both arms at the same exact time, [the cortices] fight over who gets that control. I can see that inhibition.”
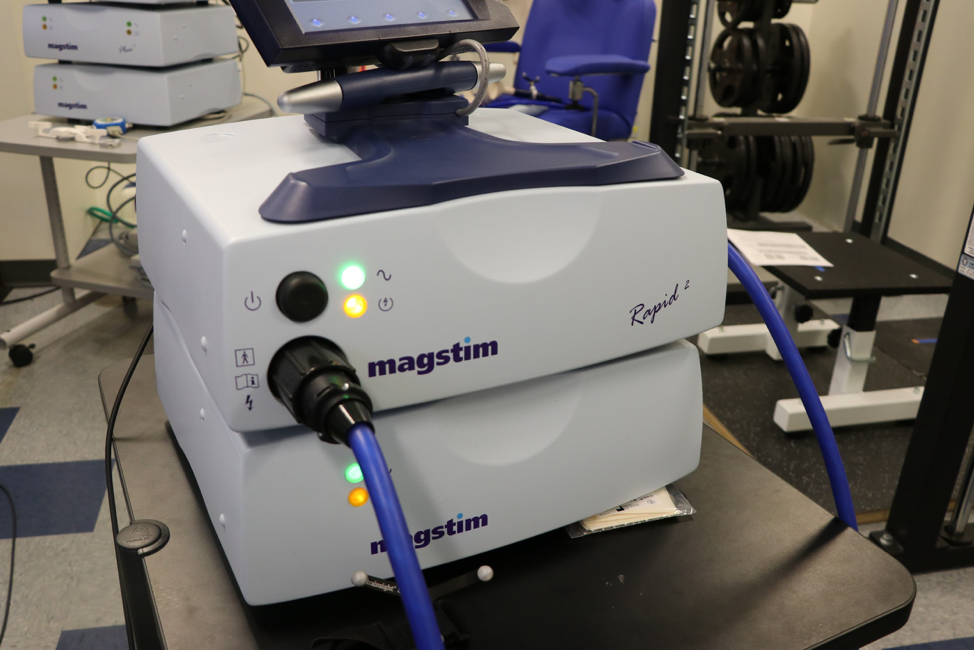
Next, she places EMG sensors on the person’s body, using henna dye to make sure she puts the signals on the same spot for every test. EMG sensors are similar to what you would wear during an EKG heart test. They measure muscle activity and then display it in wavelengths on a chart.

Beethe can also use Neuronavigation, a system using infrared markers to map out the shape and size of someone’s brain to then display it on a computer screen. She places invisible infrared dots all around the subject’s brain, creating a computerized image of their head. When she sends a current through that person’s brain, a red arrow appears on the screen to show her exactly where on the head she sent the current. This allows her to send it in the same spot every time during her testing.
She once used a swim cap and sharpie markers, but this new method is much more precise.
This precision is important because the area of the brain that controls arm muscular movement is an exact one, and she wants to get it right each time to ensure her tests are accurate and consistent.
“I’m finding what we call the [motor cortex] hot spot. Every single muscle in the brain has a spot that we track,” she said. “The name of the game is getting the ball in the basket.”
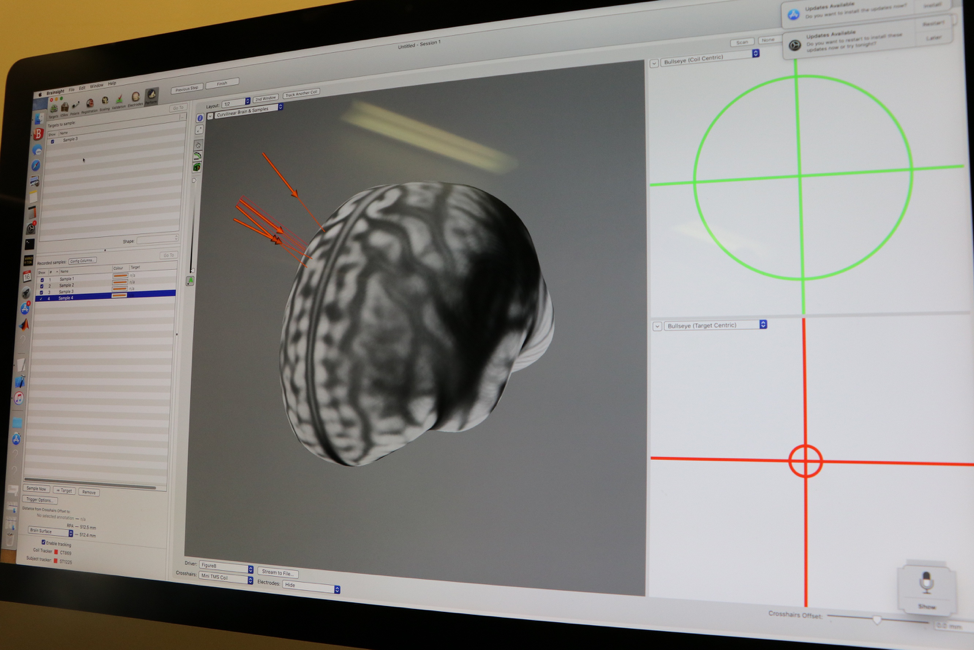
Beethe compares the neurological signals between different arm contractions. She asks her test subjects to contract one or two arms at a time to see how large those wavelengths are.
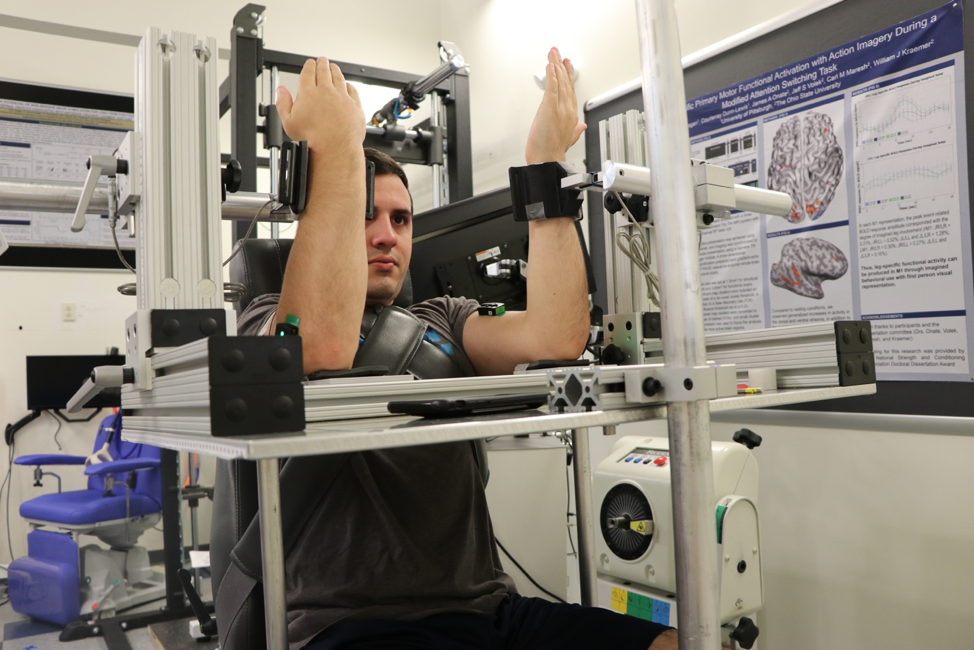
Beethe then sends an electric current through the brain to one arm, while asking the subject to contract their other arm. This allows her to see if there’s any inhibition, interruption or pause as the neurological signals, each coming from a different hemisphere, cross paths. While muscle movement seems second nature, it actually involves an intricate web of neurological pathways and signals. She wants to see if the two neurological signals inhibit each other, which would cause the contractions to be weaker or more difficult.

The tests do require a lot of work. Beethe performs seven sessions with each subject to ensure she has enough data. Subjects have to be careful they don’t use their abs, jaw or legs to help contract their arm, because that would result in unreliable contraction data and without accounting for any inhibitions.
When lifting with your arms, you may notice your jaw, abs or legs clench. That means you have extra muscles helping to add force to your lift. If Beethe’s subjects use an extra muscle to strengthen the contraction, the brain wouldn’t have to send as strong of a signal to the arms, thus preventing a possible inhibition or interruption between the signals sent from the two hemispheres. This could skew Beethe’s data.
Beethe, along with doctoral student Felix Proessl, under the guidance of Dr. Shawn Flanagan are also using the TMS in the lab’s current study with the Department of Defense. They are measuring the effects of sleep restriction, calorie restriction and physical exertion on military soldiers. She is measuring how the lack of sleep and food impact the strength of the neurological signals sent from the brain to move muscles.
“The brain changes how it organizes actions based on what we’re doing and our whole entire environment,” Beethe said.
The technology and research at NMRL helps everyone from physical therapists to everyday trainers understand those pathways more, leading to optimized performance.