By Hana Ahmed, Brittany Edelmann and Daphne Yao
Medill Reports
Near light-speed collisions of streams of subatomic particles at the Fermi National Accelerator Laboratory near Batavia have helped identify several of the 17 known building blocks of matter, leading to continuing and newfound discoveries about the universe.
New Neutrino?
One experiment focuses on neutrino physics. But first, what is a neutrino?
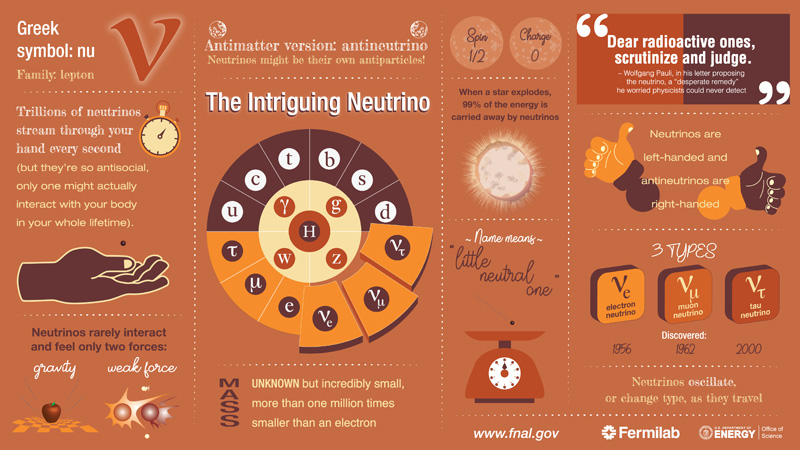
(Artist/Creator: Diana Brandonisio for Fermilab)
Neutrinos are nearly undetectable particles with no charge and masses so tiny that they “don’t like to interact with anything,” said Fermilab postdoctoral research associate David Caratelli. That makes neutrinos “difficult to catch and study,” even though trillions of them are flowing through each of us every second, and leads to more and more questions about them, he says. So then how do we learn about these particles, elusive even though they are everywhere?
Neutrinos are related to the forces that produce fusion energy in stars including our sun, helping to power the sun.
In 2015, a Nobel Prize in Physics was awarded for the discovery of neutrino oscillations, otherwise known as the ability of a neutrino to change from one type to another, showing that neutrinos have at least some mass. This discovery was a “key element that goes beyond what our current theories predict,” Caratelli said of a breakthrough made by Takaaki Kajita and Arthur B. McDonald.
But, as most good research does, the discovery prompted scientists to ask even more questions. What if there is something more beyond the neutrino oscillations that we are currently unaware of? That led to the development of further neutrino research at Fermilab.
To answer this question, Caratelli focuses on trying to find out if there are more kinds of neutrinos than the three that are currently known. To follow the trail, large detectors at different distances are filled with liquid argon and the electronics inside are used to take photos of infrequent neutrino interactions and measure the changes in the type of neutrinos, Caratelli explained. In one case, to optimize the chances of detecting interactions, the distance spans several hundred miles to an underground detector in Minnesota.
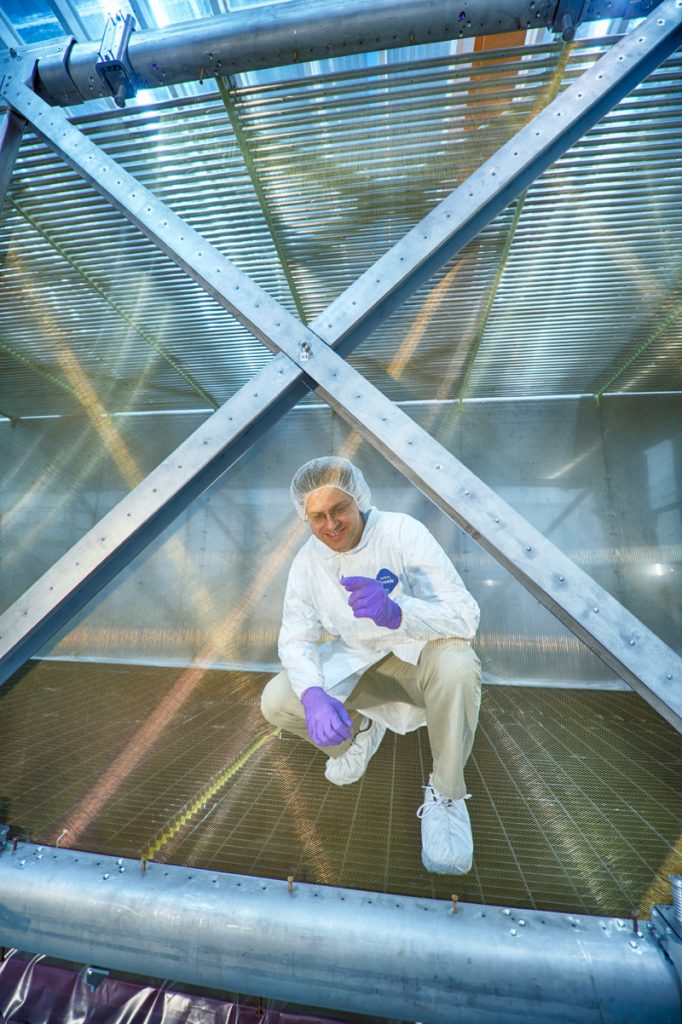
The detector that Caratelli works with, known as MicroBooNE, has been collecting data for about five years and is “starting to provide first answers to this question,” Caratelli said. This detector is located on the Booster neutrino beamline at Fermilab.
There are still many questions in relation to neutrinos because of how difficult they are to study. These questions are very connected to the deepest questions of particle physics related to how our universe came to be, and why our universe is dominated by matter versus anti-matter, Caretlli said.
Muon g-2: Finding the Monsters
For a while, we understood electrons, protons and neutrons to be the smallest building blocks of the universe. Physicists now know that particles are, in fact, much more numerous. Protons and neutrons are made up of smaller, still more fundamental particles, while electrons are their own fundamental particles. Altogether, 17 fundamental particles have been discovered so far as part of the Standard Model of matter.
New research regarding muons — small, electron-like fundamental particles that only survive for 2 millionths of a second before they decay — recently made headlines around the world. Their behavior suggests an 18th particle because the muon shows sensitivity to forces that were not accounted for by current theories. The news reached over 3.5 billion people earlier this year.

At Fermilab, where the goal is to understand these fundamental building blocks of nature, scientists have been working on the Muon g-2 experiment for about 10 years. “I’m having this fundamental realization now,” said Chris Polly, a physicist at Fermilab and the co-spokesperson for the Muon g-2 project. “When you’re going into particle physics, you’re lucky if you get to do (a new) experiment every 10 years.”
Scientists at the Fermilab were especially lucky this time. Years of experimenting and theorizing have led to results that Polly says “strongly suggest there must be an 18th particle out there,” meaning the possibility of discovering a brand-new fundamental particle.

(Photographer: Reidar Hahn for Fermilab)
In February, over 170 collaborating scientists gathered for the unveiling of results from the decadelong project. Scrutinizing muons within their milliseconds’ lifetime in particle accelerators boosted by superconducting magnets at Fermilab, scientists found these particles behaved differently than what the Standard Model predicted.

The Standard Model is a well-established theory of particle physics that describes the interactions between elementary particles like muons and electrons. These elementary particles are constantly surrounded by other particles that pop in and out of existence, changing the behavior of the particle being measured. Nearly 36 years after the framing of the Standard Model, scientists are challenging this commonly accepted theory.
Particles that have an electric charge, like muons, naturally generate an internal magnetic field of their own, known as the magnetic moment. Scientists sought to measure the strength of this magnetic moment by observing the rate at which these particles spin in a lab-generated external magnetic field. The magnetic moment should be proportional to a constant, known as the g-factor. According to previous theories of quantum physics, the value of the g-factor should always be two.
Yet, in the Muon g-2 experiment, scientists found evidence that the g-factor is greater than two. This discovery implies either the existence of a new particle or something incorrect about the way scientists currently understand interactions between elementary particles.
When the Muon g-2 experiment found that the g-factor deviated from the accepted value of two, scientists posited that this is likely because there is a new particle changing the behavior of the muon.
Fermilab’s results agree well with previous experiments conducted at Brookhaven National Laboratory in 2001. But the experiments at Fermilab, using near-frictionless superconducting magnets, confirmed the results repeatedly. Polly also took part in the Brookhaven experiments as a graduate student. The results of the Fermilab Muon g-2 experiments are more precise and show more of a difference. The experimental average now significantly deviates from the theoretical value of 2, with only a 1 in 40,000 chance that you could get such results are purely coincidental. As such, results from the Muon g-2 experiments will surely spark a new round of theoretical speculation of what the universe is fundamentally like.
“Maybe there are monsters lurking out there,” Polly said.
Hana Ahmed, Brittany Edelmann and Daphne Yao are health and science reporters at Medill. You can follow them on Twitter at @Hana_Ahmed045, @brittedelmann, @daphnecyao.